
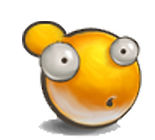
SB lab
RNA editing, Genome stability, Cancer & Protein Quality Control
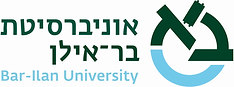

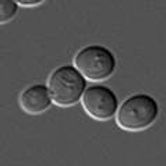
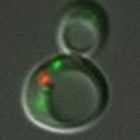
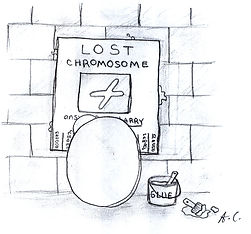
Our Laboratory:



​
The fundamental rationale that underlies our lab research is that for highly conserved biological pathways, results from model organisms can greatly facilitate functional discovery in humans. We use the the bakers' yeast Saccharomyces cerevisiae as a model organisms, for the understanding of complex mammalian systems, with an emphasis on human cancer, protein quality control and primarly on RNA editing.
​
In one line of research created we screened the yeast mutant collections for genome instability phenotype. The functional distribution of the identified genes suggests the existence of multiple unexpected genome integrity pathways. Many of the identified genes encode for proteins that participate in the biosynthesis of iron-sulfur (Fe-S) proteins, and proteasome subunits. The work in our lab is aimed at understanding the mechanisms leading to genome instability in these pathways.
​
In another major line of research, we try to uncover new determinants that contribute to protein miss-folding and to understand how the protein quality control (PQC) machinery prevents their accumulation. Such accumulation is one of the hallmarks of cancer and multiple syndromes, including neurodegenerative disorders like Alzheimer’s. We do this by employing a wide variety of high-throughput screening techniques in yeast (some developed in our lab), complemented by dedicated cell biological, genetic, and biological follow-ups.
In the past four years we entered a new research area, as we became interested in the Adenosine deaminases acting on RNA (ADAR) protein family, which modify individual nucleobases within RNA molecules. When editing occurs within mRNAs, it can recode specific codons, leading to changes in protein structure and function. We try to determine if similarly, to DNA mutations, RNA editing can also serve as a source of proteotoxic stress. Another question that we want to address is how RNA editing enzymes carry out their activity in regions of dsRNA formation. The determinants for such activity are currently unclear and defining them can be used to edit particular genes by utilizing the endogenous ADARs. In contrast to humans, yeast cells do not edit RNA, but the genetics of their apparatus for protein quality control are well understood. Utilizing our lab expertise in harnessing the yeast for the study of fundamental biological problems, we successfully expressed the ADAR enzymes originated from human cells in yeast. Computational analyses of RNAseq data obtained from these cells, clearly show that ADAR enzymes are active in our model system. Furthermore, we already created a selection-neutral in vivo system to investigate the determinants of RNA editing enzymes substrate preference.
​

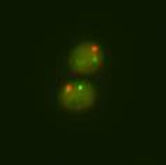
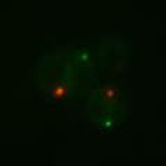
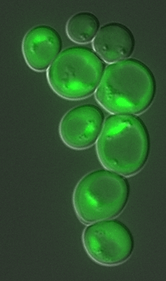

